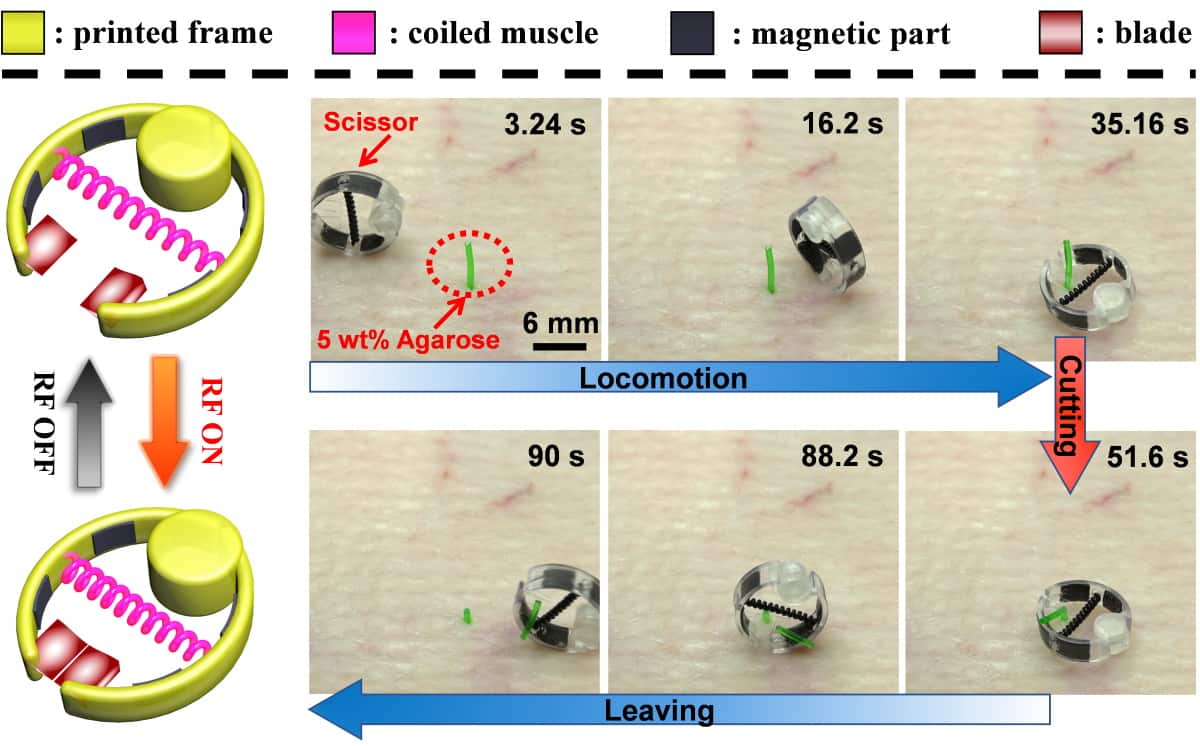
Wireless miniature soft robots that can safely navigate confined spaces in response to an external magnetic field are highly desirable for medical applications such as minimally invasive surgery. In addition to locomotion, soft robots must be able to perform other complex tasks if they are to replace conventional rigid tools. Such tasks, however, require high force or torque outputs that softer materials alone cannot produce. Instead, a combination of different materials, ingenious mechanics and structural optimization is required to store and release the large amounts of mechanical energy needed to wirelessly perform complex surgical tasks.
To achieve this, scientists from the Max Planck Institute for Intelligent Systems in Stuttgart have developed a small-scale coiled muscle actuator that relies on tunable mechanical properties and radiofrequency magnetic heating to perform surgical tasks that require a high force output and work capacity, including suturing, cutting, drilling and clamping tissues. The researchers describe the capabilities of the miniature soft medical robots in Science Advances.
Coiled artificial muscles
Artificial muscles in soft robotics aim to reproduce the mechanical versatility of natural muscles – contracting, expanding, rotating or bending to efficiently generate motion or perform tasks. To fabricate the muscle actuator, the group used a high-strength nylon fibre as the stiff muscle core and covered it with a polymer layer to insulate it from external mechanical and thermal stimuli.
Between the core and the outer cover, the researchers embedded a high-toughness resin matrix layer containing superparamagnetic Fe3O4 nanoparticles and graphene oxide platelets. These magnetic nanoparticles produce heat when exposed to radiofrequencies, actuating the muscle core and enhancing the mechanical strength of the actuator. Finally, they twisted the resulting structure into a coil to form artificial muscle with an approximate diameter of 1 mm.
The researchers applied an external radiofrequency magnetic field to wirelessly heat the embedded nanoparticles. The artificial coiled muscle then contracts if the fibre ends are free but cannot rotate, or twists if one end of the fibre is fixed and the rest can rotate. The team systematically optimized the concentration of graphene oxide platelets and thickness of the active layer to achieve an actuation force and work capacity of roughly 3.1 N and 3.5 J/g, respectively. These values are more than five and four orders of magnitude greater than those previously reported for magnetic soft actuators.
“Thanks to the muscle actuators integrated to wireless soft medical devices, we can now achieve surgical functions that require high force outputs, such as suturing, cutting, clamping and drilling. Such functions have not been possible so far for wireless soft medical devices,” explain senior authors Wenqi Hu and Metin Sitti.
Performing surgery with soft robots
To fully exploit the potential wireless applications of their coiled artificial muscles, the researchers devised five proof-of-concept surgical scenarios to demonstrate their utility.

First, they sutured a previously punctured ex vivo pig skin sample by attaching two nylon fibre bars and a cylindrical permanent magnet to the ends of the coiled muscle. By using precise magnetic field control and leveraging the large output force of the coiled muscle, the team showed how the device magnetically reorients itself to suture around the wound and contracts to close it.
Second, they 3D printed a circular polymer frame and embedded magnetic microparticles in a silicone elastomer distributed throughout the frame circumference. This enabled the frame to perform rolling locomotion and positioning using a teleoperated magnet. In the demonstration, the researchers used the force generated by contracting the coiled muscle to cut a synthetic tissue-like material in half using two glass blades located at the opening of the frame.
Next, to test the torque performance, the team leveraged the high energy-release of the artificial coiled muscle and combined it with a 3D-printed driller frame and screw to wirelessly penetrate a synthetic tissue-like material by applying radiofrequency magnetic heating to untwist the coil.
The researchers then engineered a bistable clamper that uses coiled muscle contraction and stored mechanical energy to rapidly pinch tissues during surgical operations. The continuous contraction of the coiled muscle drives the clamper from a stable to an unstable energy state. This triggers the snap-through, amplifying the output force of the coiled muscle up to 14 N – allowing the researchers to clamp an ex vivo chicken tissue wound.
Finally, to showcase the versatility of multiple coiled muscles, the team coated an additional polymeric layer with a spatially varying magnetization profile in the coil, and linked four coiled muscles together using softer connectors to amplify the magnetic deformation. This new structural configuration allowed the multi-linked coils to “walk” forwards, backwards or move axially by combining magnetic torque and magnetic gradients. This could enable the device to perform more complex manipulations as well as the high force output demonstrations.
“Our new approach extends the capabilities of wireless medical soft robots to surgical applications. As next steps, we will demonstrate such functions in small animal surgeries in in vivo conditions,” Hu and Sitti tell Physics World.